Laboratory of terahertz science and devices
Department of Electrical Engineering and Computer Science
Tokyo University of Agriculture and Technology
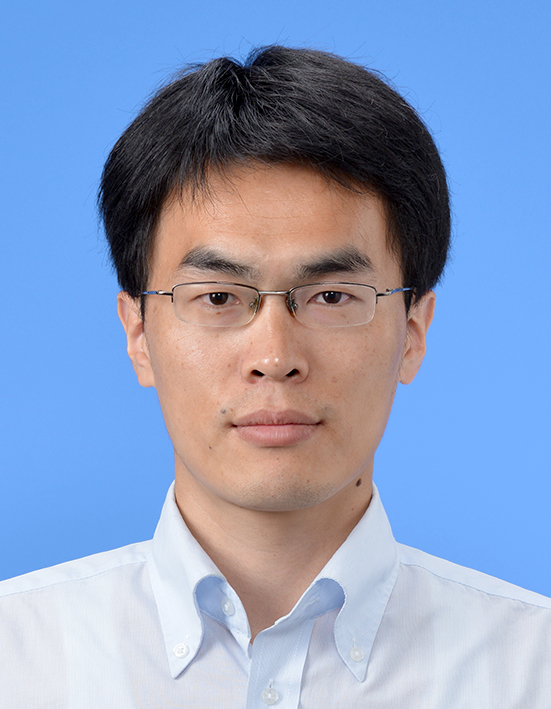
zhangya[@]go.tuat.ac.jp
Associate Prof. Ya Zhang
Academic Background
Undergraduate: 9 /2002~7 /2006 Precision Instruments and Mechanics, Tsinghua University (Beijing, China)
Master: 9 /2006~7 /2008 Optical engineering, Tsinghua University (Beijing, China)
Doctor: 10 /2011~9 /2014 Electrical engineering and information systems, University of Tokyo (Tokyo, Japan)
Research Experiences
8/2008~7/2011 Beijing Oriental Institute of Metrology and Measurement, Researcher of Metrology
12/2014~3/2016 University of Tokyo, Project Researcher
4/2016~ 2018.3 University of Tokyo, Project Research Associate
4/2018~ now Tokyo University of Agriculture and Technology, Associate Professor
Research Interests

Terahertz (THz) radiation generally refers to the spectral region that lies between the microwave and the infrared (IR) regions, spanning a frequency range of 0.1 THz to 10 THz, and showing a dual characteristic of both microwaves and light waves. THz spectroscopy is a powerful tool for characterizing electronic properties and vibronic excitations in various kinds of solids, liquids, and gases and has been extensively used not only for basic science but also for industrial applications.
The research in Zhang lab focuses on the physics and applications of semiconductor micro/nano structures in the THz frequency range. We use THz wave as a powerful tool to explore the novel physics and device principles in semiconductor micro/nanostructures, such as quantum dots, single molecules, and etc. We also develop novel high sensitivity and fast THz sensors by using MEMS/NEMS resonators. Device physics and nonlinear behavior of MEMS resonators are also our research interests.
Applications for master/doctor courses are highly welcome! Currently we have a FLOuRISH fellowship that could provide a full scholarship to outstanding PhD students. All PhD students will be recommended for the application. Other than that, there are also many other chances to get financial supports from scholarships, RA/TA, and etc.
Research Topics
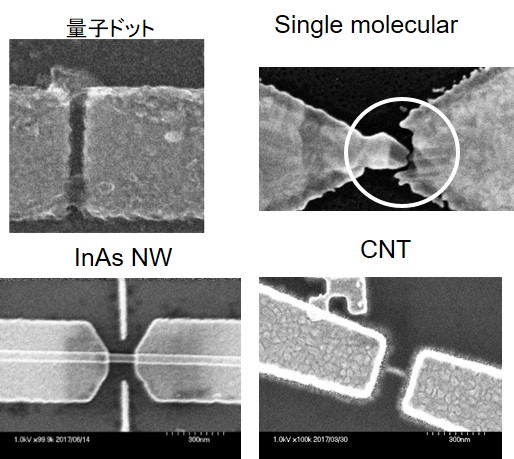
Nanoscale terahertz spectroscopy
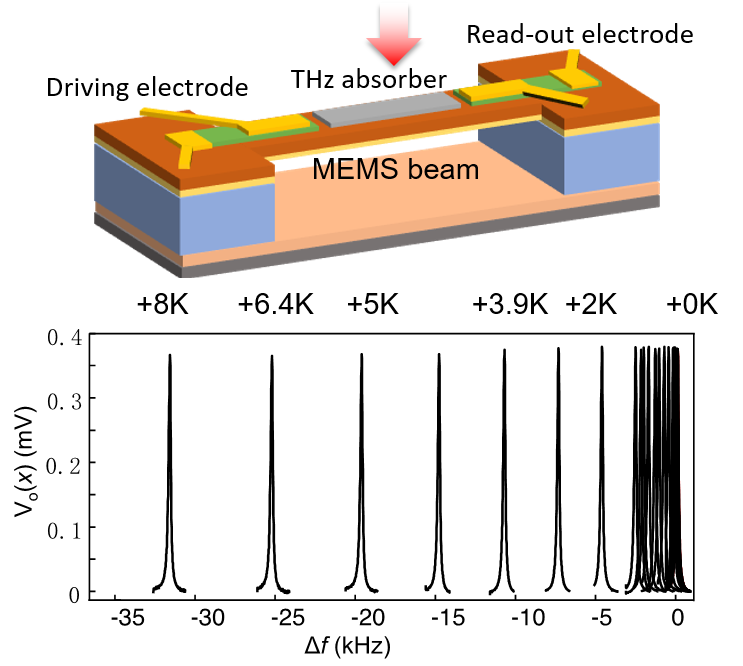
MEMS terahertz sensor
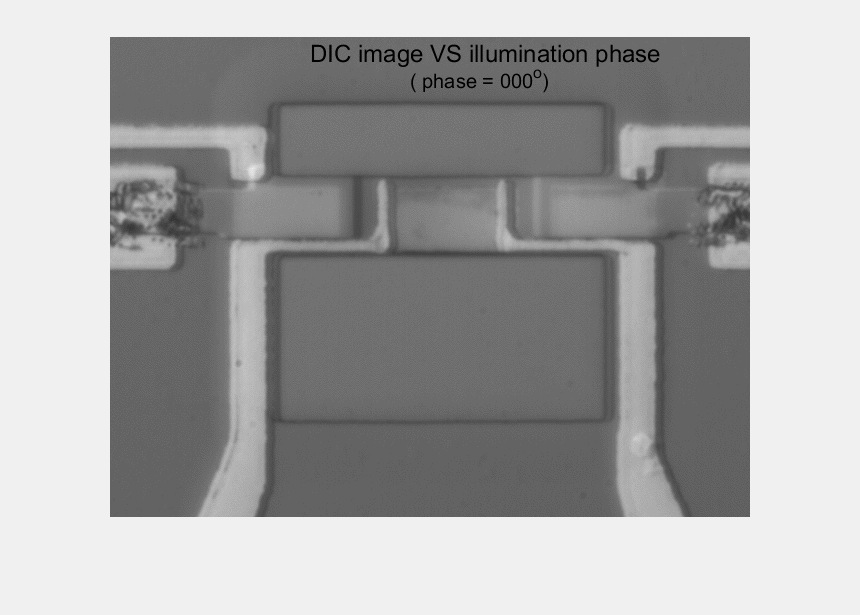
MEMS motion monitoring with a DIC microscope
Our research covers a broad range of THz devices and physics. Some recent research will be introduced below. If you would like to have quick understanding of our research, you may refer to the following two review papers we have published.
C. Li, Y. Zhang, and K. Hirakawa. Terahertz detectors using microelectromechanical system resonators. Sensors 23(13), 5938. 2023年7月
Zhang, Ya, Shaoqing Du, and Kazuhiko Hirakawa. Deep-nanometer-scale terahertz spectroscopy using a transistor geometry with metal nanogap electrodes. Light: Advanced Manufacturing 2.4 (2021): 460-472.
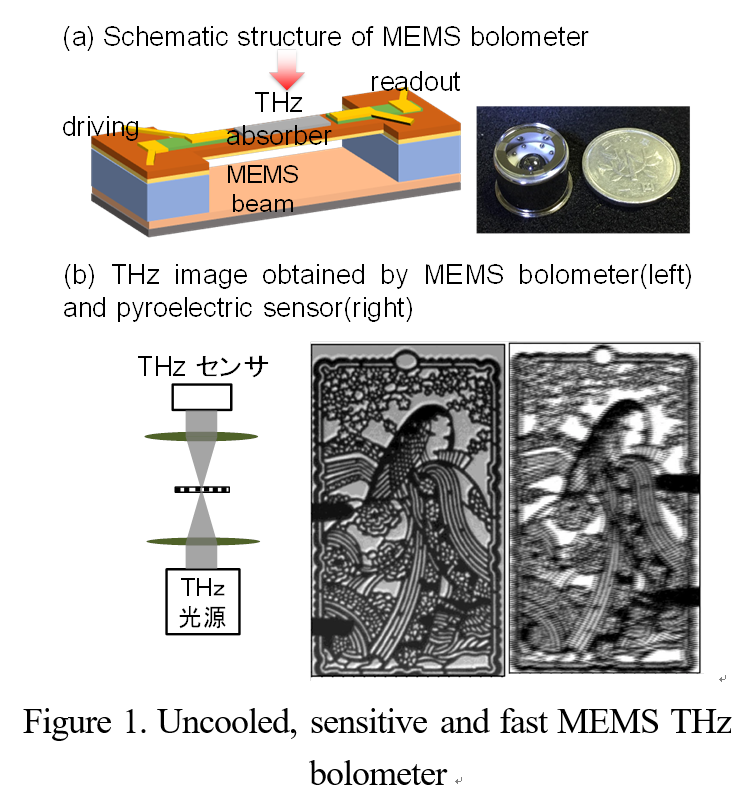
Terahertz detection using microelectromechanical system (MEMS) resonators
MEMS resonator is a microstructure with mechanical degrees of freedom and fabricated using semiconductor microfabrication technology (see Fig. 1a). The applicant has developed a compact, sensitive, and fast THz bolometric detector using a GaAs doubly clamped MEMS beam resonator. MEMS resonator detects the input THz radiation as a shift in the resonance frequency and works as a very sensitive THz detector1,2. This unprecedented temperature sensitivity of the MEMS resonator is advantageous not only for realizing high sensitivity, with noise equivalent power (NEP) as low as ~20 pW/√Hz, but also for achieving a high-detection speed at the order of 10 kHz, which is more than 100 times faster than other uncooled THz thermal detectors (see Fig. 1b).
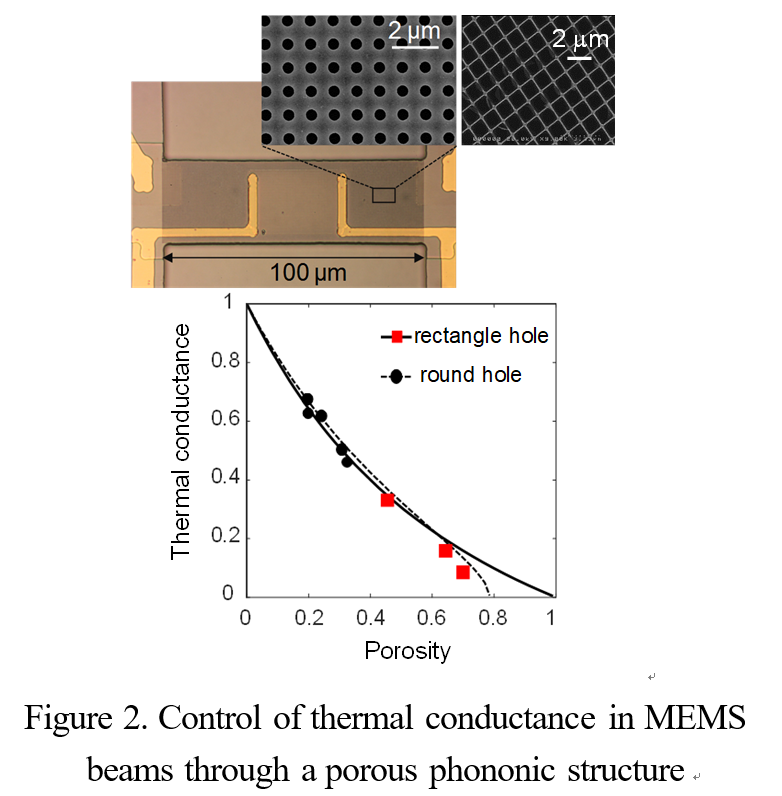
Improving the sensitivity of MEMS bolometer through porous phonon structures
Generally, the thermal sensitivity of a bolometer is inversely proportional to the thermal conductance of the structure. To increase the sensitivity of bolometers, thermal conductance is usually strongly reduced in the device design, which however will greatly increase the thermal time constant and reduce the operation speed of the device. To address this issue, the applicant has introduced a porous phononic structure in the MEMS bolometer4 (see Fig. 2). The porous structure reduces not only the thermal conductance but also the heat capacity of the MEMS beam. Therefore, the thermal sensitivity is improved without significantly degrading the response speed of the MEMS bolometer. The applicant has successfully fabricated MEMS beams with very high porosity (proportion of pore volume) of 0.7, which reduces the thermal conductivity of the beam by 90% and increases the thermal sensitivity of the MEMS radiometer by more than 10 times5.
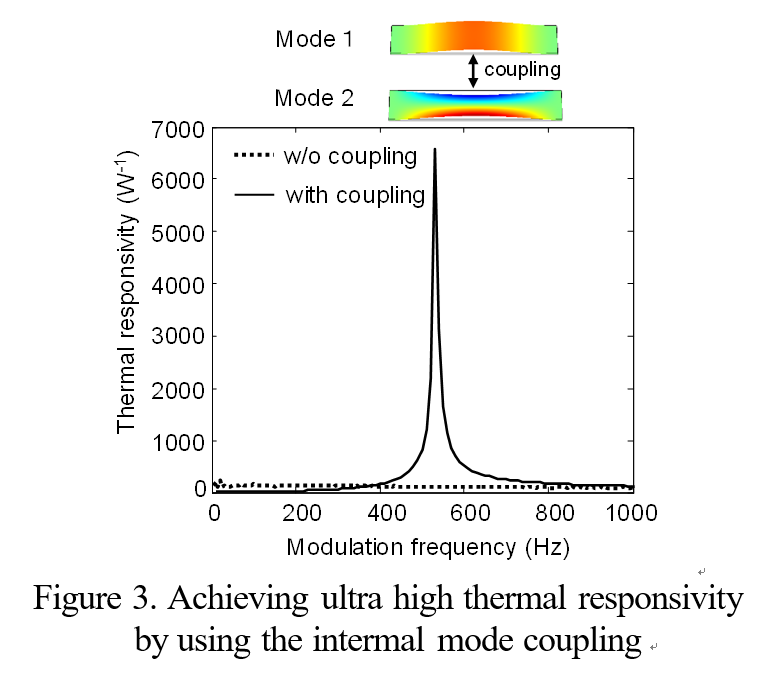
Giant enhancement in the thermal response by internal mode coupling
The applicant has found a giant enhancement in the thermal responsivity of MEMS resonators by using the internal mode coupling effect. This is achieved by coupling the fundamental bending mode with the fundamental torsional mode of the MEMS beam resonators through the cubic Duffing nonlinearity. In the mode coupling regime, when the input heat to the MEMS resonators is modulated at a particular frequency, the resonance frequency shift caused by heating can be enhanced by almost two orders of magnitude6 (see Fig.3). The observed effect is promising for realizing high-sensitivity THz detection, and also other sensing applications with MEMS resonators.
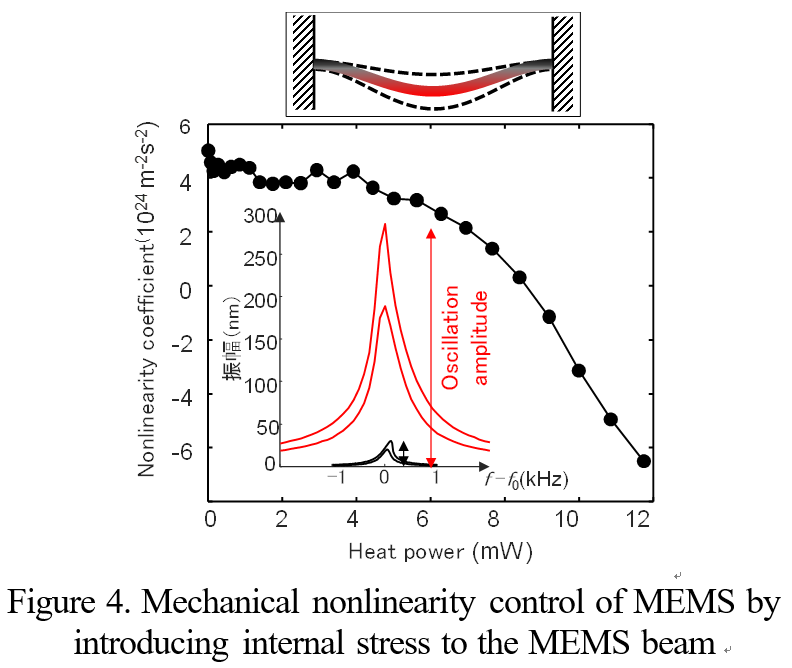
Improving MEMS output signals through mechanical nonlinear control
The mechanical nonlinearity is a common issue that limits the signal-to-noise ratio of MEMS resonator. The MEMS beam resonator has a hardening nonlinearity, i.e., as resonance frequency increases with the oscillation amplitude. To maintain a stable oscillation condition, MEMS resonators generally works in the linear oscillation regime that has very small oscillation amplitude of ~10 nm. Here, it has been found that the mechanical nonlinearity of the MEMS resonators can be significantly reduced by introducing internal strain to the MEMS beam. The decrease in the mechanical nonlinearity is originated from the bending of the MEMS beam, which gives a negative nonlinearity term and hence compensates the total nonlinearity. With the thermal tuning effect, the MEMS resonator can maintain a very large quasi linear oscillation amplitude of ~ 300nm (see Fig. 4), which is ~10 times larger than the linear oscillation range without the control of nonlinearity7,8. The observed effect is very promising for improve the output signal level of MEMS devices.
References
1. Y. Zhang, Y. Watanabe, S. Hosono, N. Nagai, and K. Hirakawa, Appl. Phys. Lett. 108163503 (2016).
2. Y. Zhang, S. Hosono, N. Nagai, S. H. Song, and K. Hirakawa, J. Appl. Phys. 125151602 (2019).
3. I. Morohashi, Y. Zhang, B. Qiu, Y. Irimajiri, N. Sekine, K. Hirakawa, and I. Hosako, Journal of Infrared, Millimeter, and Terahertz Waves 41675 (2020).
4. Y. Zhang, B. Qiu, N. Nagai, M. Nomura, S. Volz, and K. Hirakawa, AIP Advances9085102 (2019).
5. R. Yamamoto, A. Kojima, N. Koshida, I. Morohashi, K. Hirakawa, and Y. Zhang, Sensors225109 (2022).
6. Y. Zhang, R. Kondo, B. Qiu, X. Liu, and K. Hirakawa, Phys. Rev.Appl. 1401400119 (2020).
7. Y. Zhang, Y. Yoshioka, M. Iimori, B. Qiu, X. Liu, and K. Hirakawa, Appl. Phys. Lett. 119163503 (2021)
8. C. Li, B.Q. Qiu, Y. Yoshioka, K. Hirakawa, and Y. Zhang. Physical Review Applied, 19, 024025 (2023).